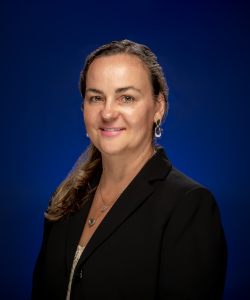
Welcome
Dear Students,
Welcome to the Health Sciences and Human Ecology Department! We are thrilled to have you join us in your pursuit of knowledge and passion for improving the health and well-being of our community. Whether you are interested in Environmental Health Science, Nutrition Science and Dietetics, Health Services Administration, Public Health, or one of our graduate programs, we are here to support and guide you on your academic journey.
Many of our programs have earned external accreditations, ensuring you receive an exceptional education and are well-prepared for an exciting career.
At the Health Sciences and Human Ecology Department, we take pride in serving a diverse student body, including many first-generation college students. We believe in providing practical training opportunities to enhance your learning experience. This includes internships, supervised practice experiences, and field placements in local hospitals, public health facilities, and various profit or nonprofit organizations. These hands-on experiences will empower you to apply your knowledge and skills in real-world settings.
Our faculty consists of 13 full-time professors and numerous part-time faculty members who are experts in their respective fields. Many of our faculty members work in various organizations, allowing our students to benefit from their firsthand experiences. We are proud of our faculty's commitment to diversity, equity, and inclusion, which is evident in their publications, research endeavors, and service to the community. Their dedication has garnered awards and national and international recognitions.
As you embark on your academic journey with us, we encourage you to explore your interests, ask questions, and make the most of the resources available to you. Our department is here to support you every step of the way.
Once again, welcome to the Health Sciences and Human Ecology Department. We look forward to helping you achieve your goals and contribute to the health and well-being of our community.
Sincerely,
Dr. Terezie Tolar-Peterson, Professor and Chair
Mission Statement
Our mission of the Department is to prepare highly competent professionals who are well prepared in the disciplines of public health including environmental health science, health care management and administration, nutrition and food sciences, and public health education, and to maintain the department as a public health leader within the University's service area. Graduates will exhibit broad health science knowledge, applied technical and human skills, clear personal and social values, civic commitment to the community, and a desire for lifelong learning and achievement. To this end, we are committed to achieving excellence in undergraduate and graduate education by being at the forefront of scientific advancement and professional practice in the areas of research, teaching, and service consistent with the philosophy and goals of the California State University System, the San Bernardino campus, and the College of Natural Sciences. We help students prepare for jobs in local health departments, community organizations, health services, schools, hospitals, industry, tribal health, and many other sites. Our students may also pursue graduate programs in research, epidemiology, physician assistant, nursing, occupational and respiratory therapies, and other allied health careers.
We are Ranked
- CSUSB ranked among best graduate schools in the nation by U.S. News & World Report
- # 150 Public Health California State University--San Bernardino - Best Health Schools - US News
- # 10 for most affordable universities - CSUSB News
- See more CSUSB rankings!
Administrative Support Coordinator II
Jennifer Strei currently serves as the Administrative Support Coordinator for the department of Health Science and Human Ecology. She enjoys supporting all faculty, students, and staff, and is adept at figuring out how to best serve their needs. With her caring and reliable nature, Jennifer takes pride in being a valuable team player, providing excellent service with a happy disposition. She eagerly looks forward to meeting everyone and contributing to the department's success.
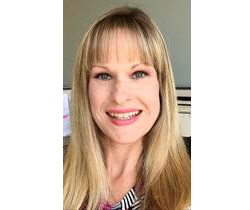
Administrative Support Assistant II
Carrie Hartshorn is the Administrative Support Assistant for the Department of Health Science and Human Ecology. Carrie assists all students, faculty and guests who come into the HSCI Department Office. She likes to promote positivity within the department office, and help students in any way so that they may accomplish their goals here at CSUSB. She feels that if there is anything she can do to help students achieve their goals, she will go above and beyond for them.
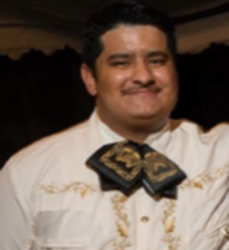
Student Assistant
“Luis Trejo is the Student Assistant for the Department of Health Science and Human Ecology. In our department, Luis helps all faculty, students, and staff however possible. From helping students navigate through our department resources to cleaning instructional labs, Luis does a little bit of everything. He is majoring in Biology to eventually pursue a career in Medicine. Luis actively volunteers at a local community hospital to help others and to get a big picture of all roles in the medical field. We are excited to see what Luis accomplishes in his time with us at CSUSB.
Luis Says he loves the interaction he has with students and all members of the department. He says that being social is a big part of his life, especially as a college student. If you ever run into him in our office or on campus, always feel free to spark up a conversation.”